EXPERT TALK: power electronics unleashing sustainable potential in the energy transition
The energy transition towards a carbon-neutral future is a pressing global challenge. In this endeavour, Power Electronics (PE) plays a pivotal role in driving the optimisation and efficiency of energy conversion systems. Through continuous innovation and technological advancements, PE is revolutionising how we harness and utilise renewable energy sources.
This Expert Talk, written by EnergyVille / KU Leuven Professor Wilmar Martinez, explains why power electronics stands at the forefront of the energy transition, driving sustainability and efficiency.
Power Electronics Applications
PE solutions are tailored to specific applications to ensure optimal and cost-effective operation. For instance, the flash charging of electric buses at bus stops reduces the need for large batteries and allows for more passengers. Sophisticated digital algorithms can transform battery energy storage systems into virtual synchronous generators, providing essential grid support. Power Electronics is also crucial in rail electrification, providing different AC voltages, frequencies, and DC voltage. Furthermore, applications like STATCOM and Power Quality Filters stabilise current and voltage fluctuations, increasing the productivity of industrial facilities and facilitating the integration of variable renewable power plants. Moreover, PE is an enabler for Solar and Wind Energies; power optimisers, central, string and micro-inverters have been used in solar plants to enable flexibility and higher energy yields, while HVDC solutions can enable high-efficiency power transfer from offshore wind to onshore or between countries, enhancing grid reliability.
Efficiency at the Core
Efficiency is the cornerstone of PE, enabling optimal energy conversion. Currently, at least 70% of all electrical energy is processed by a power electronics device, proving its importance in the energy landscape. Every conversion step in the power system incurs losses, and PE strives to minimise these losses. Moreover, suppose an average of 95% weighted efficiency (conservative case as there are converters with efficiencies of 80% or less in the field) is assumed in a system with only one conversion stage and considering a consumption of around 3000 TWh/year in Europe. In that case, at least 105 TWh/year are estimated to be lost in losses due to power conversion. Then a quick calculation of how much that represents in money can be done with the weighted electricity prices of each country, resulting in billions of euros lost because of this. Nevertheless, this estimation is done ONLY for one conversion stage.
For instance, offshore wind power generated in the North Sea requires a modular multilevel converter (MMC) to convert AC power into High Voltage DC (HVDC). Onshore, another MMC is needed to convert HVDC back into AC power. Subsequently, multiple conversion steps in the transmission and distribution system further step down the voltage. This step could be done in the near future with the concept of solid-state transformers (compact alternatives to conventional 50Hz transformers). Finally, onboard chargers and dedicated installations can charge electric vehicles at the end-user level, even allowing for phone charging within the vehicle. Fig. 1 shows the representation of these conversion stages.
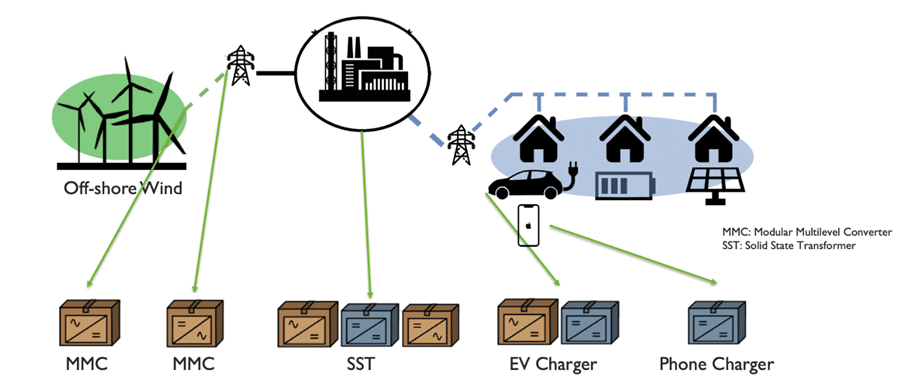
Fig. 1. Conversion stages and converters per stage to supply a phone from offshore wind power. Figure redrawn from [1]
Inside buildings, a similar situation happens. An interesting example is the power distribution in the two buildings of EnergyVille, as Fig. 2 depicts. First of all, it is visible a Bipolar DC grid that is interconnected between the two buildings. This is important, as it is perhaps the first DC connection between buildings in Belgium, possible due to the regulatory sandbox for energy. Moreover, the amount of conversion stages in the building is evident and reaffirms the argument of efficiency and losses due to power conversion in our buildings. Fig. 3 shows a 3D representation of the current status of the LVDC installation in EnergyVille 1.
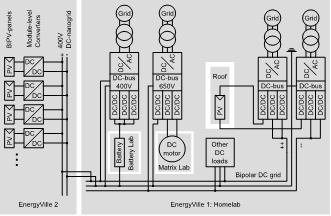
Fig. 2. Conversion stages in the two buildings of EnergyVille
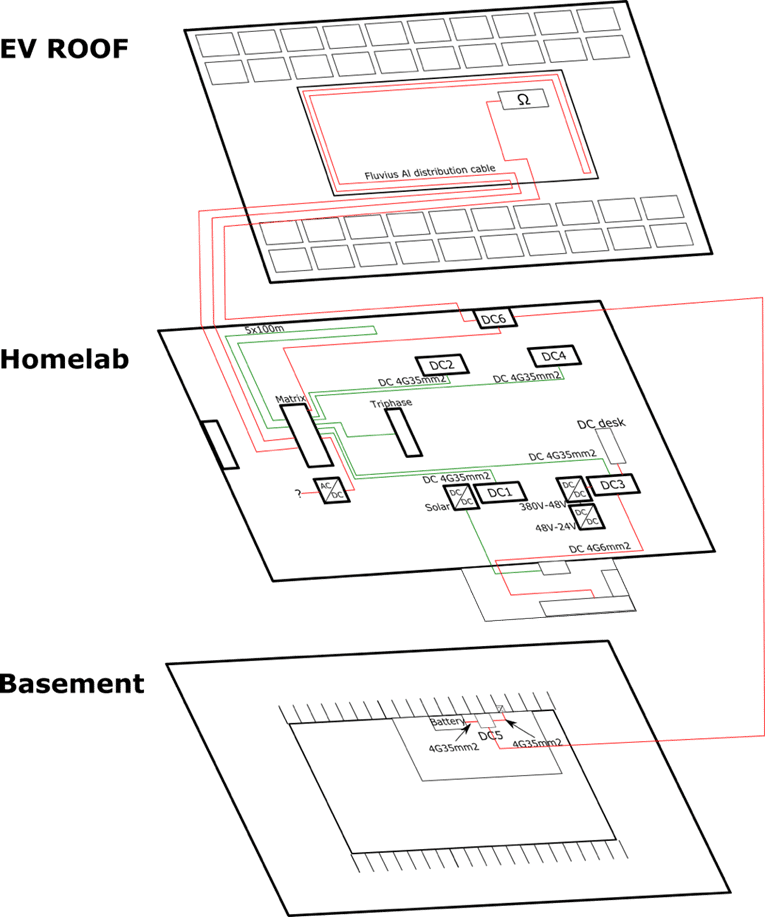
Fig. 3. Conversion stages in EnergyVille 1
A testament to the vital role of research in power electronics in achieving a highly efficient power system. On top of the fact that in this power system, power electronics is crucial to taking over the role of keeping the grid stable, as conventional power plants are starting to be shut down in the coming decades.
Advancements in Power Electronics
PE is continuously evolving, driven by advancements in various areas. Power semiconductors, the heart of converters, are continuously improving. Silicon carbide (SiC) power transistors, for example, offer higher efficiency, faster response times, and reduced size compared to traditional silicon devices. This leads to more efficient and compact power converters that respond rapidly to grid changes. Advanced digital controllers have also enabled precise system control, performing millions of calculations per second. The evolution of digital technologies, such as edge and cloud solutions, augmented reality, machine learning, and digital-twin technologies, further enhances system controllability, data analysis, decision-making, and serviceability.
Unlocking Potential through Data
Data-driven insights and predictive maintenance techniques have become invaluable assets within PE. Through advanced analytics and machine learning algorithms, power electronics systems can monitor performance, detect anomalies, and proactively address potential failures. Predictive maintenance optimises system operation, reduces downtime, and extends the lifespan of critical components. Moreover, integrating artificial intelligence (AI) brings intelligent decision-making and adaptive control strategies, further enhancing PE capabilities.
AI-Driven Design Automation
AI is revolutionising the design process of power electronics systems. By leveraging vast data, AI algorithms optimise system architectures, component selection, and control strategies. This streamlined approach expedites the design process, accelerates time to market, and facilitates the development of highly reliable and efficient solutions.
Future of Power Electronics: Key Technological Aspects
While PE has already made significant contributions to the energy sector, there is room for further development towards a carbon-neutral energy system. Key technological aspects requiring urgent attention are:
- Semiconductors and System Design: New power semiconductor devices, including wide bandgap devices like silicon carbide, are needed for higher voltage, current, and efficiency applications. The advanced system design must address challenges in layout, EMI/EMC2 design, power quality, and impedance shaping strategies to ensure grid compatibility and stability. Integration at different levels demands new concepts to address the challenges of thermal, insulation, testing, manufacturing, and cost reduction.
- New Applications: The increased use of PE converters challenges power system stability. Methods for analysing, assessing, and mitigating problems arising from the integration of thousands of power converters need to be developed.
Conclusions
Power Electronics stands at the forefront of the energy transition, driving sustainability and efficiency. PE transforms how we convert and utilise energy by harnessing the power of SiC and GaN devices, advanced magnetic components, predictive maintenance techniques, and AI-driven design automation. Through its continuous evolution and commitment to sustainability, PE is unlocking the true potential of renewable energy sources. As we move forward, the synergy between PE and emerging technologies will pave the way for a greener and more sustainable future. With PE processing at least 3/4 of electrical energy, its role in achieving an efficient and sustainable energy landscape cannot be overstated.
[1]: Ballestín-Fuertes, J.; Muñoz-Cruzado-Alba, J.; Sanz-Osorio, J.F.; Laporta-Puyal, E. Role of Wide Bandgap Materials in Power Electronics for Smart Grids Applications. Electronics 2021, 10, 677. https://doi.org/10.3390/electronics10060677
[2]: EMI/EMC: ElectroMagnetic Interference / ElectroMagnetic Compatibility. Referring to the noise challenges that will bring due to fast switching and transients in power converters.