Expert Talk: Comparing one Joule to another
We all like it to be comfortably warm, not too warm though, so thermal comfort is important! To realise the energy transition in an efficient manner it’s crucial that the right energy sources are used for the right applications and that the right Joule is used for the right Joule. This Expert Talk zooms in on what possible energy sources can be used to realise sustainable heating and cooling in the built environment and for industry.
Figures and numbers
We all like it to be comfortably warm, not too warm though, so thermal comfort is important! Current figures show that today we are mainly trying to achieve this by heating and cooling the indoor climate. In Europe, heating is expected to decrease (by 30% by 2030) and cooling to significantly increase (by 72% by 2030). In European households, space heating and the production of domestic hot water represent 79% of the final energy use (2,240 TWh in EU-2016). The tertiary sector adds an additional 1630 TWh/year for building heating and cooling. In industry, 70.6% of energy use goes to space heating and process heat (2250 TWh in EU-2016). In buildings, 75% of the energy used for heating and cooling is generated from fossil fuels (in industry this is almost 100%), while only 18% originates from renewable energy sources (11% represented by biomass, especially wood, and 7% by sunlight, wind and hydropower via electricity in heat pumps or resistance heating). It goes without saying that decarbonisation of the production of heat and cold can contribute significantly to a reduction in greenhouse gas emissions and an increase in the share of renewable and residual energy sources.
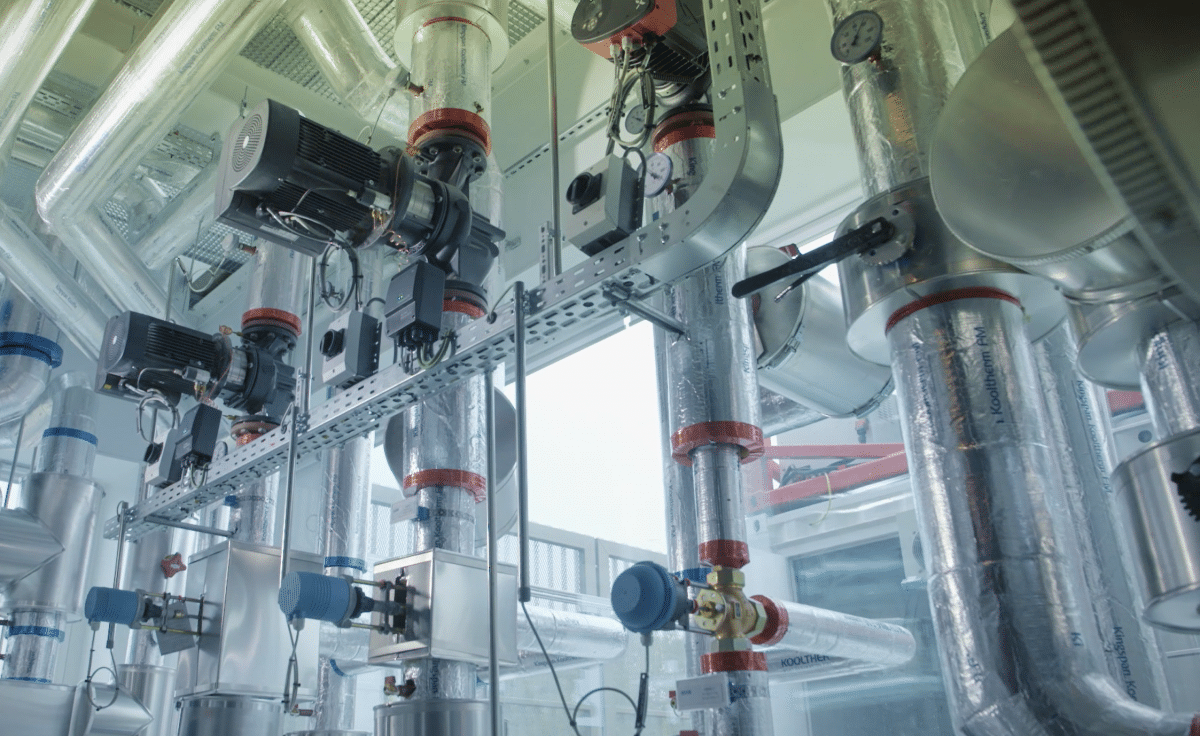
Comparing one Joule to another
Some background. According to the first law of thermodynamics, energy is never lost, so we cannot destroy energy or create extra. Yet something can change in energy conversions, namely the quality of energy. This is where the term ‘exergy’ comes in. With thermal energy, for example, the temperature at which heat/cold is available determines the quality and therefore also the usefulness of an energy exchange.
The thermodynamic definition of Exergy is “the maximum amount of work obtainable from the system when it is brought into equilibrium with the environment.” Work is the highest quality form of energy transfer. The exergy factor is defined as the work potential of a system divided by the amount of heat that can be obtained from the same system.
For heat, it depends on the temperature at which the heat transfer takes place (T) and the temperature of the environment (T0), see figure 1. Heat at ambient temperature has no work potential, in other words the exergy factor is zero. With increasing temperature, the exergy factor increases (and approaches one at infinitely high temperature). At temperatures lower than the ambient temperature the exergy factor rises very sharply and approaches infinity at zero Kelvin. Cold at temperatures below the ambient temperature therefore has a large work potential, or vice versa: it takes a lot of work to reach or maintain very low temperatures.
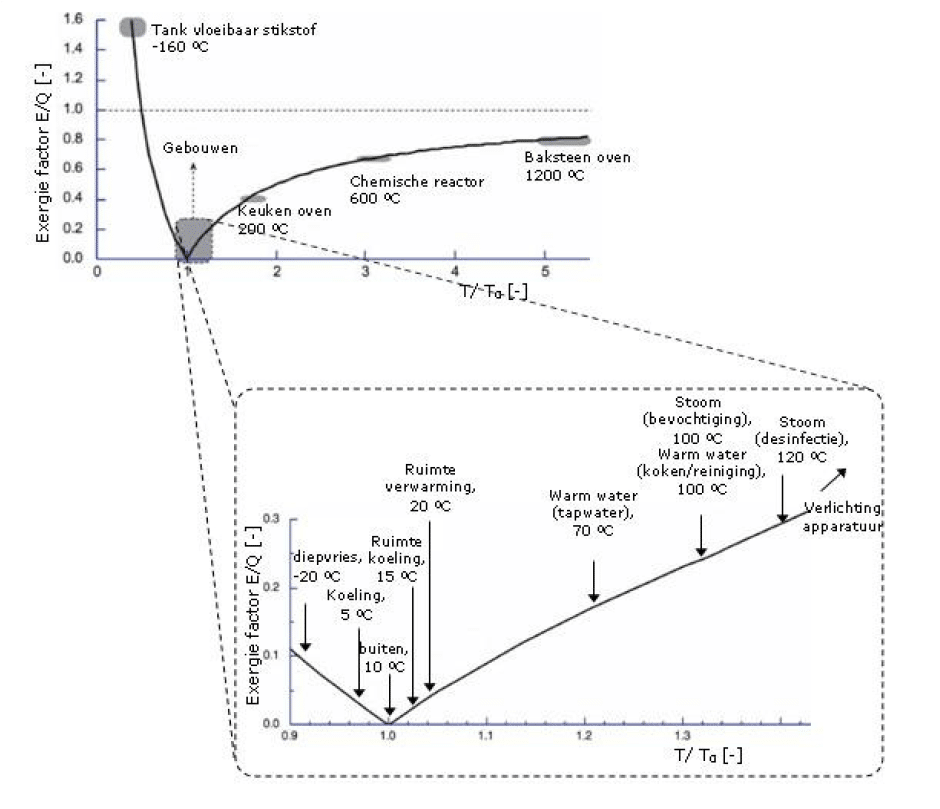
Figure 1: Exergy Factor as a function of T/T0, with below application from the built environment
(Source: De Vaan 2007).
As mentioned above, energy is never lost, but the quality can change, and it decreases in processes that occur spontaneously. According to the second law of thermodynamics, the quality of the total amount of energy will either remain the same or decrease. A heat flow from a low temperature system to a high temperature system can never occur spontaneously. To achieve this, we need work or high-quality heat, such as used in a heat pump.
Heating in the built environment
Potential for improvement. Heat flows in the built environment are usually close to ambient temperature and therefore have a low exergy factor and relatively little exergy content. Theoretically, these heat flows can be realized with little work. In practice, however, energy sources with a high exergy factor are often used here, such as natural gas, which when combusted leads to flame temperatures above 1000°C. The switch from natural gas to (green, i.e. generated from renewable sources) hydrogen will result in a carbon-free variant, but with the even higher flame temperatures for hydrogen combustion, the exergy loss when used for space heating is awfully large. After all, the quality of the energy used is many times higher than the quality of the energy required. While the energy efficiency of the gas boiler is almost 100%, the exergy efficiency for the heat transferred to the room is only 5%. This means there is great potential for improvement.
Efficient and renewable. A better choice are technologies for the production of low-temperature heat, which score high in terms of efficiency (both energetic and exergetic) and use renewable energy sources. Solar collectors and heat pumps (with air, soil, groundwater, surface water or low-temperature residual heat as a source) meet this requirement and are already being used in individual buildings today. Solar collectors are heat exchangers that convert direct and diffuse sunlight into warm water, but of course only when the sun is shining. Heat pumps use a small amount of high-exergetic (green) electricity to upgrade low-quality heat (renewable and available in the environment) to usable heat.
Example: H2020-project hybridGEOTABS
The performance of a heat pump is all the better when the source is at a higher temperature and the heat emission system at a lower temperature. GEOTABS systems, the combination of geothermal heat pumps (GEO) and thermally activated building structures (TABS), are a nice answer to this. The undergound has a relatively constant temperature of about 10°C at a depth between 20 and 100 m, which is higher than the outside air temperature in winter. TABS have a large heat-exchanging surface area, so that the temperature for heating can be relatively low. In winter, the heat pump in a GEOTABS system therefore works with a high COP (coefficient of performance = heat released per unit of electrical input). But even in summer we can cool with a very high EER (energy efficiency rate = cold (or heat absorbed) per unit of electrical input) because after a winter the underground is sufficiently cooled so that direct cooling via a heat exchanger is possible. As a result, heat is injected into the soil again and the underground is heated up again after the summer so that the heat pump can again operate at a high COP in the winter. The underground therefore serves as a seasonal storage. If the temperature of the underground increases too much during the summer (with a high cooling demand), it is possible to switch to active cooling after a while, which means that the heat pump is switched to function as a chiller, see figure 2.
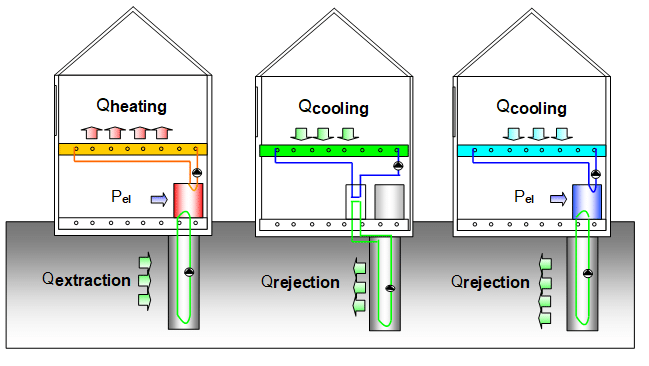
Figure 2: The heat flows in a GEOTABS system for 3 applications: heating, direct cooling, active cooling
The TABS typically consist of a concrete structure in which water-carrying pipes are embedded, resulting in high thermal inertia. As a result, a TABS system cannot react quickly to disturbances in the building and we have to anticipate as much as possible, as well as provide a supplementary system to respond to fast disturbances, hence hybridGEOTABS. The control of this hybrid system requires a different approach than the conventional rule-based room thermostat (Rule Based Control – RBC). For this we have developed white-box model-based predictive control (MPC) within the EU-H2020-hybridGEOTABS project.
White-box refers to the controller model that is physically based. MPC solves an optimisation problem over a chosen time horizon by minimizing a cost function, for example the energy use of the building, by optimally choosing the control variables of the building, for example the frequency of the pump. This involves using the white-box model of the system and predictions of the disturbances. In addition, a number of constraints, such as a minimum and maximum zone temperature, can be imposed. The inherent flexibility of thermal systems (typically heat pumps in combination with thermal energy storage) can also be exploited via the same MPC. This is done by responding to variable energy prices, where the cost function is the operational cost. The results are very promising. Figure 3 shows that the savings in electricity consumption for the heat pump in a GEOTABS building when using MPC is significant compared to operation with RBC.
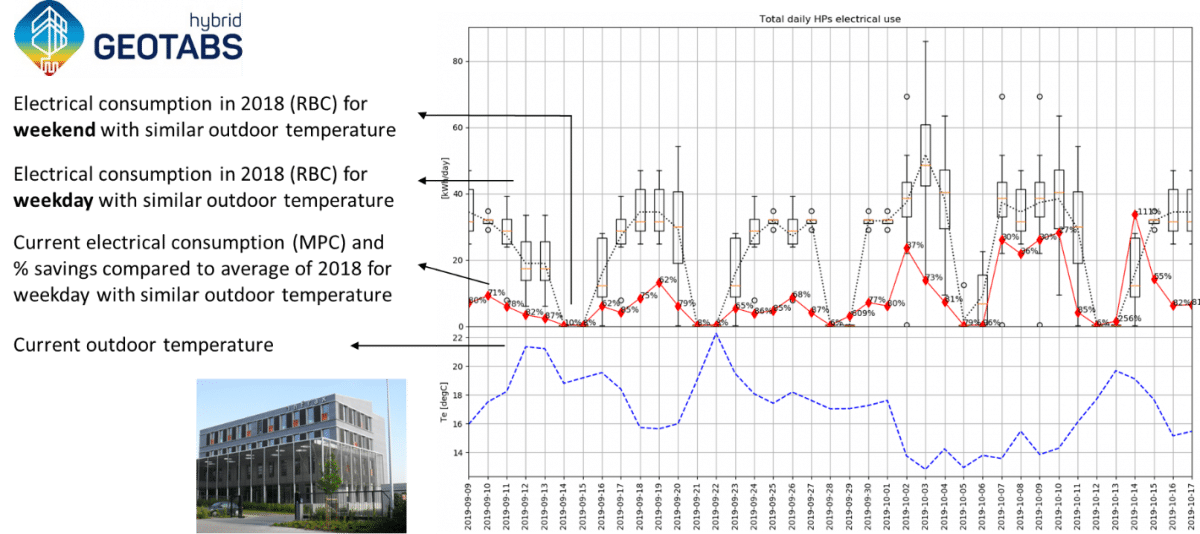
Figure 3: Electricity consumption for heat pumps in the GEOTABS Fluvius-Boydens office building (Dilbeek): comparison between RBC (Rule Based Control) and MPC (Model Predictive Control).
Collectively via clusters of buildings. The collective use of these technologies can achieve a nice synergy through extensive system integration. In sufficiently densely built city districts, for example, solar energy could be stored in a central thermal seasonal storage unit in summer, and then distributed from there via a thermal network to meet demand in winter, whether or not locally upgraded via heat pumps. It goes without saying that this requires smart control that can also use flexibility to further increase the share of renewable energy and/or residual heat. After all, heat pumps form the link between electricity demand and heat production.
Example: H2020-project STORM
A good example of this is the Mijnwater project in Heerlen (NL). The water from the old flooded coal mine Oranje Nassau III is used as a sustainable heat and cold source. The water that is pumped from five sources is transported via an underground exchange station and pipe network to residential areas and 9 large office buildings. The further extension to the entire urban area of Heerlen is currently being carried out. The mines and the network are responsible for the supply of both low temperature heat (28°C) and high temperature cold (16°C). The heat is pumped up from flooded mine galleries at a depth of about 700 m. Water in the higher mining plateaus is used for cooling. Furthermore, the residual heat from buildings (e.g. through cooling of a datacenter) is used as a source for heating other buildings. Heat pumps in the buildings then upgrade the heat to the correct temperature level to be used in the heat emission systems.
Within the H2020 project STORM, coordinated by EnergyVille, an artificial intelligence-based thermal grid control system was developed and demonstrated. The purpose of this controller is to optimise the operation of the network through active management of thermal demand.
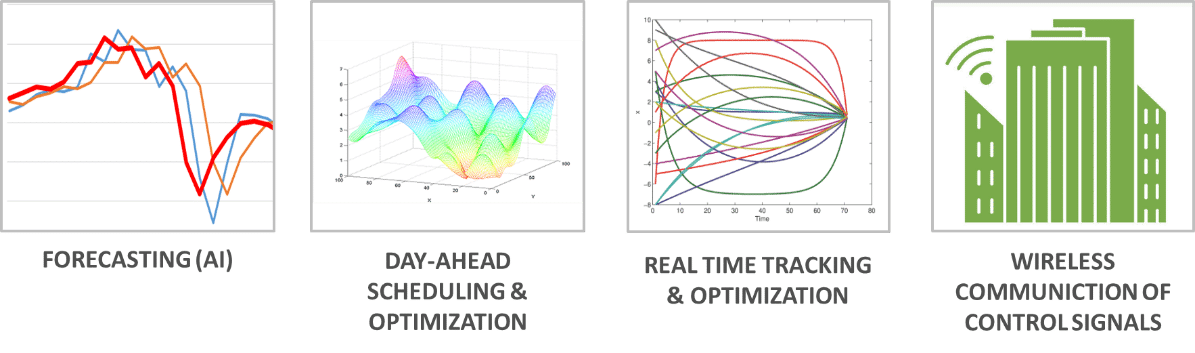
Figure 4: basic building blocks of the STORM Energy District Controller
The project focused on flattening peaks in heat demand by using energy storage in the building mass. By temporarily reducing the heat demand of a building, peaks in the heat network can be reduced, which offers advantages in various areas. After all, through peak reduction the capacity of the network can be increased, as the network and the heat production systems are sized for peak heat demand, which occurs only sporadically in our climate. By reducing the peaks in heat demand, more buildings can be connected to the network without additional investments in heat production capacity and/or pipes in the ground.
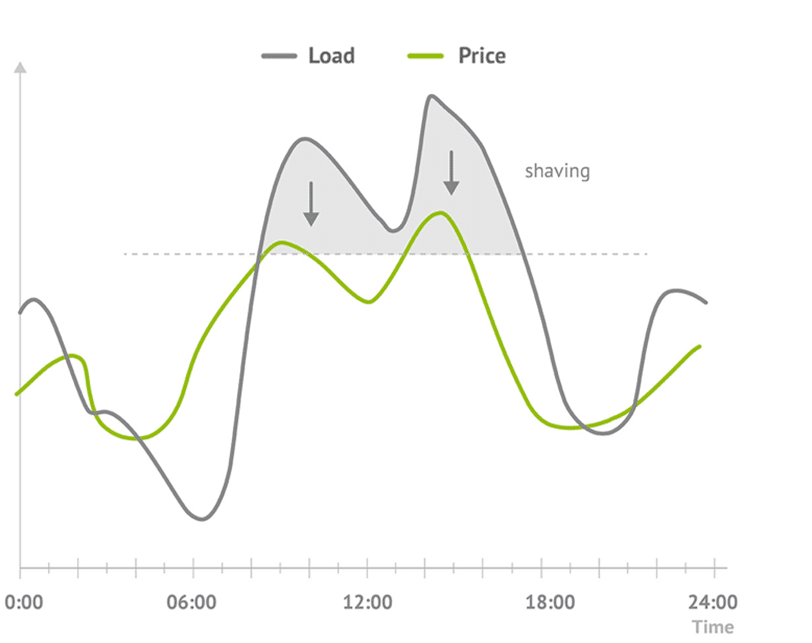
Figure 5: Example of flattening peaks in heat demand
In the STORM project, this controller was developed by EnergyVille in collaboration with the Swedish company Noda and demonstrated in two existing networks: on the one hand, a high-temperature network operated by VEAB in Sweden and, on the other hand, the above-mentioned Minewater project and network in Heerlen. The results achieved were impressive. The peaks in the heat demand were reduced by 17.3% on average and the capacity of the network was increased by 42.1%, which corresponds to approximately 48,000 additional residential units that can be connected without loss of comfort. If these extra homes are connected to this sustainable heat and cold source from the underground, this corresponds to a CO2 emission reduction of 11,2 kton/year. Within EnergyVille, we will therefore continue to focus on 4th and 5th generation thermal networks to distribute sustainable heat, aided by a thorough digitisation of thermal networks.
Heating in the industry
Residual heat. Industrial processes often lead to residual heat, which can no longer be used within the company, and is therefore often discharged into the environment (surface water or air), which leads to thermal pollution (and loss of exergy). This residual heat often has an appropriate temperature, or can be upgraded via a heat pump with a high (energetic and exergetic) efficiency to the appropriate temperature for meaningful use in buildings. Sector coupling can result in the exchange of thermal flows with a good match in exergy content for applications where supply and demand are not too far from each other.
Where this proximity cannot be invoked, residual heat could be locally upgraded to, for example, electricity, via thermoelectric materials (TEM) or via organic Rankine cycles (ORC). TEM make use of the Seebeck effect, in which an electrical potential is built across a series of semiconductors subjected to a temperature difference. The temperature difference is the driving force that allows it to operate even at relatively low temperatures, which is a strong point, in addition to low weight, no moving parts, high reliability, low maintenance and a modular and scalable character. The efficiencies are low (but that is less bad if the source is a residual flow that would otherwise be lost) and the materials are expensive. The research focuses on those aspects. Commercialisation is limited to aerospace applications. ORCs are local small-scale power plants that use a classic Rankine cycle, but with an organic working fluid to operate at lower evaporator temperatures. Numerous applications can be found in biomass CHP, geothermal energy and valorisation of residual heat with temperatures above 100°C. Electrical efficiencies drop from 10% at 100°C to 2.6-3.7% at 60°C.
Example: H2020-project Story
The construction company Beneens in Olen has invested in such an ORC installation. The company has a lot of wood waste from its own joinery and in order to further valorise this wood waste, it has invested in a wood waste incineration plant with a thermal capacity of 1.6 MW to supply heat to its own buildings and processes (the painting and drying cabins). Since the supply of waste wood is quite high and the heat demand is mainly situated in winter, the installation was expanded with an ORC with a net electrical output of 90 kW to generate electricity itself from the heat generated by the combustion of the waste wood to meet part of the electricity requirement. In order to respond to the varying demand for heat and electricity, the installation was equipped with two large thermal buffers (two water storage tanks with a capacity of 50 m³ and 20 m³ respectively). In addition, a number of modifications were made in the heat emission system of specific processes and the new office was equipped with adapted convectors so that the low-temperature residual heat from the ORC can also be partly valorised. The firm also invested in a battery to store some of the ORC’s electricity generated at night and in weekends for use during peak processes during the day. The project is one of the demonstration projects within the H2020 Story project in which the focus is on demonstrating the added value of energy storage in distribution networks.
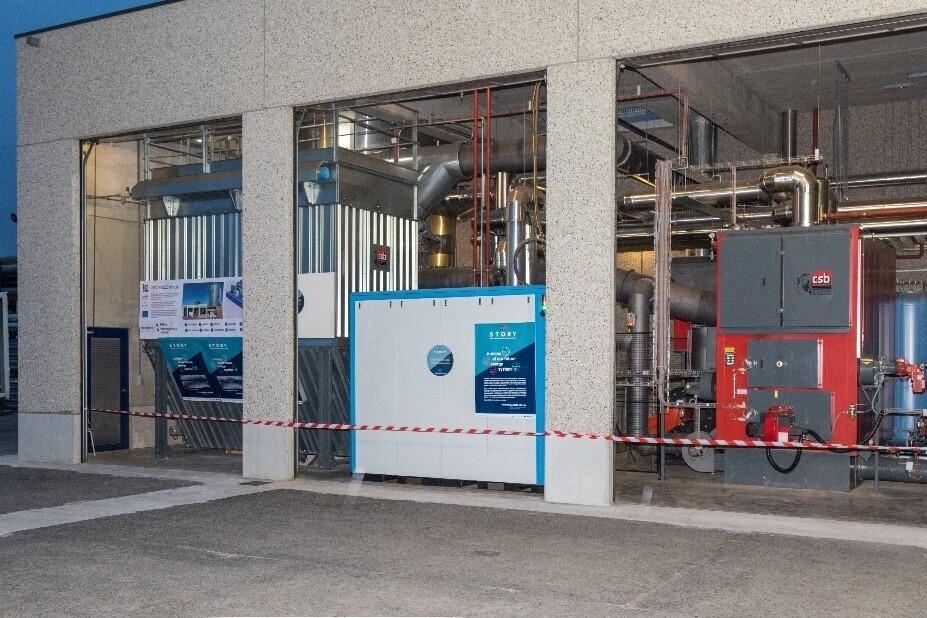
Figure 6: Waste wood incinerator (right), ORC (middle) and flue gas purification plant (left) (Company Beneens)
Heat from industrial processes. Industrial processes often require high temperature heat, which today is mainly supplied through the combustion of fossil fuels. Decarbonisation of that sector requires, in addition to as much heat recovery as possible from other processes, carbon-free fuels and electrification. Hydrogen and green gas are useful applications here and are therefore best used in these applications: high-quality heat where it is really needed.
With its research on heating and cooling in the built and industrial environment, EnergyVille follows the policy strategy as detailed in the European Green Deal, in particular:
- Focus on energy efficiency, energy circularity and use of waste heat
- Electrification where possible
- Use of sustainable molecules such as hydrogen only when necessary as a last step
EnergyVille helps shape the research and policy agenda from an integrated system approach and thus meets the demand for, among other things, thermal energy services in a sustainable way. Interested in a customised solution? Then contact us now!
Key takeaways
The energy transition will not lead to the use of a single energy source, but rather we will need a combination of multiple sources. It is crucial that the right sources are used for the right applications and that the right Joule is used for the right Joule. Exergy losses can be highly reduced by producing or valorising heat/cold of a suitable quality (and not higher). The technology exists and has the added advantage that it allows a higher use of renewable and residual energy sources, which also leads to lower CO2 emissions. Hybrid and/or collective systems allow more extensive system integration, possibly across multiple energy vectors, but require well thought-out concepts and advanced control as an integrator.
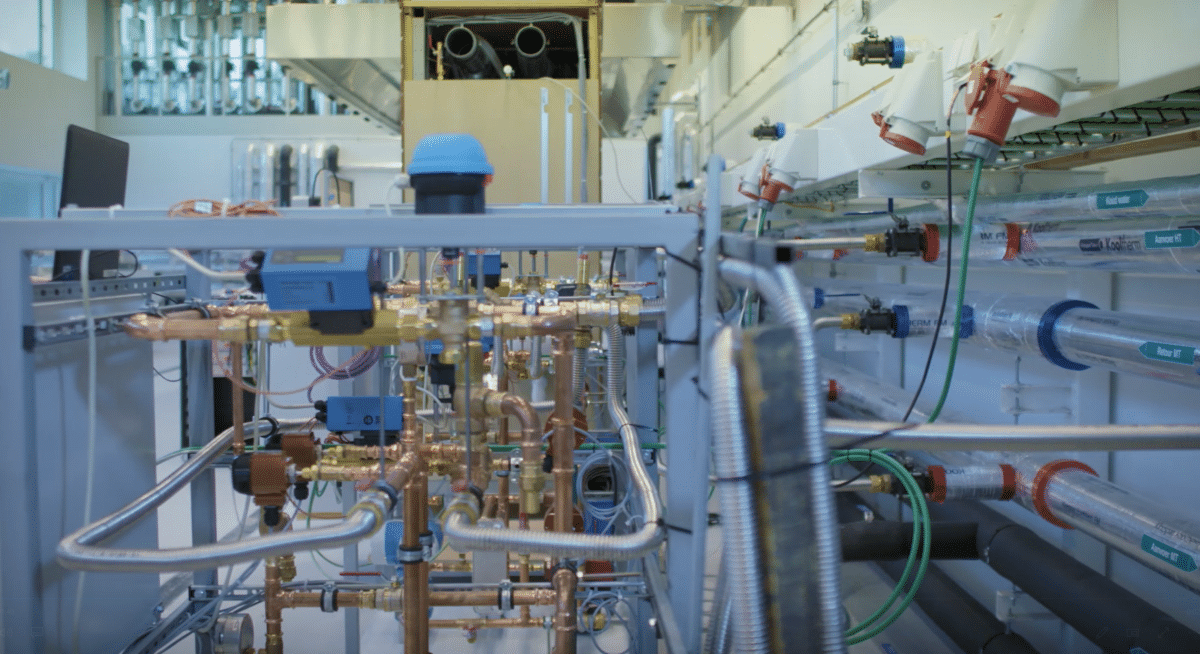
References:
- The Guardian, 2015, World set to use more energy for cooling than heating, https://www.theguardian.com/environment/2015/oct/26/cold-economy-cop21-…. [Accessed January 2020]
- European Commission “Communication from the Commission to the European Parliament, the Council, the European Economic and Social Committee and the Committee of the Regions on an EU Strategy for Heating and Cooling”, European Commission, Brussels, Tech. Rep., 2016.
- Fleiter T., Alsland R., Rehfeldt M., Steinbach J., Reiter U., Catenazzi G., Jakob M., Rutten C., Harmsen R., Dittmann F., Riveire P., Stabat P., EU profile of heating and cooling demand in 2015, Karlsruhe, Germany, Tech. Rep. 2017, http://heatroadmap.eu/output.php. [Accessed January 2020].
- Naegler T., Simon S., Klein M., Gils H.C. (2015). Quantification of the European industrial heat demand by branch and temperature level. International Journal of Energy Research, 39, 2019–2030.
- Christa De Vaan, Wat is duurzaam wonen? Een onderlinge vergelijking tussen verschillende duurzaamheidsindicatoren. Afstudeerwerk TU Eindhoven, 2007.
- EC COM(2020) 299 final ‘Powering a climate-neutral economy: an EU Strategy for Energy System Integration’.
Written by prof. Lieve Helsen and Johan Van Bael. Lieve Helsen is professor in Thermal Systems at Mechanical Engineering (EnergyVille/KU Leuven). Johan Van Bael is Project Manager and Activity Leader of optimisation of thermal energy systems at EnergyVille/VITO. Within EnergyVille both Lieve and Johan are experts in the Thermal Systems field, focusing on thermal networks, thermal energy storage and energy conversion in the built and industrial environment.